What is Mucolipidosis?
Mucolipidosis is a genetic disorder that causes problems with how our body processes certain molecules. The word 'Mucolipidosis' combines 'muco' (mucus) and 'lipid' (fat), hinting at the substances that build up in the cells of affected individuals.
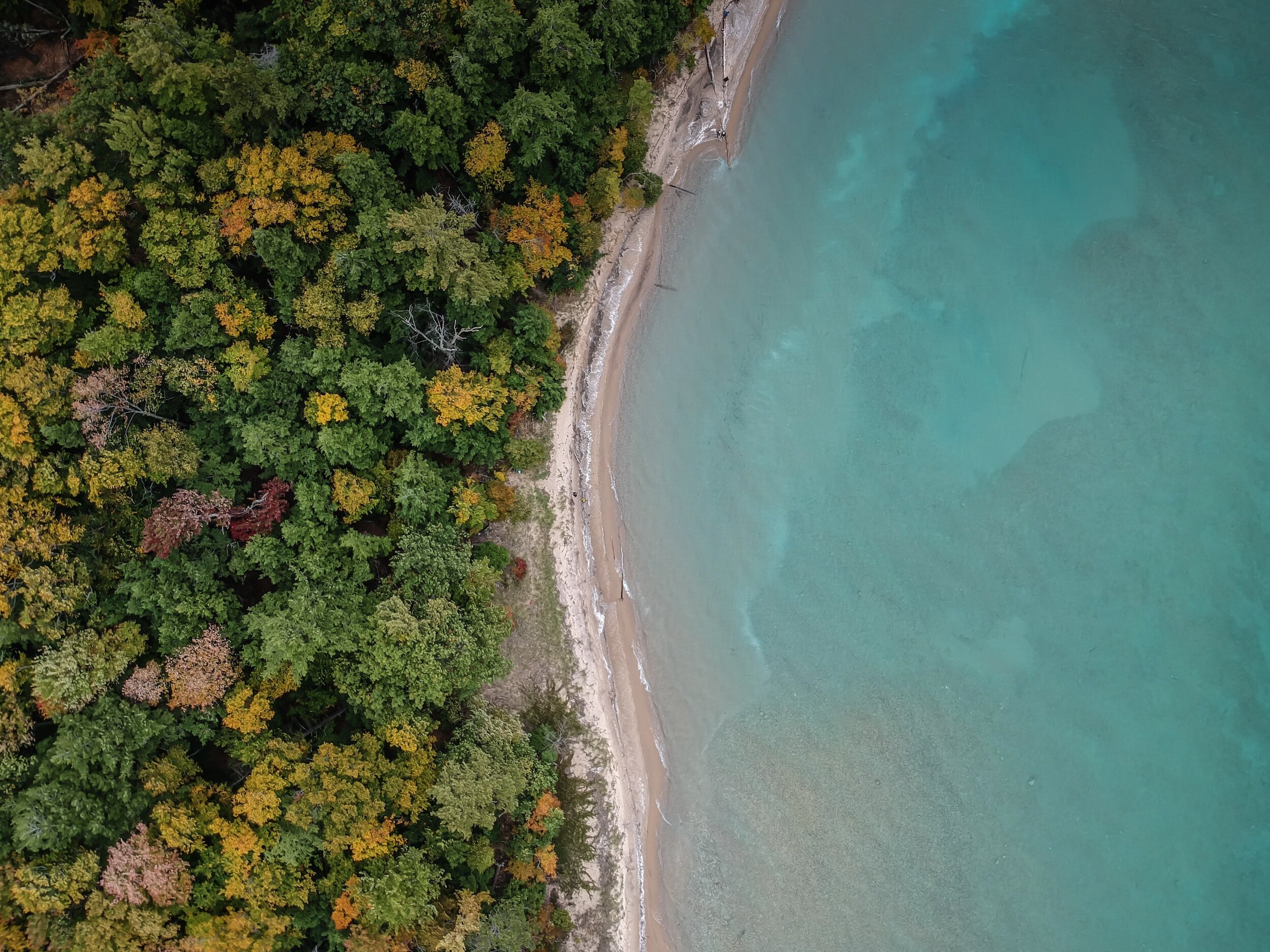
General FAQ (in layperson terms)
-
Imagine our body as a busy city, and our cells as the buildings within that city. Just as buildings produce waste that needs to be disposed of, our cells produce waste as well. To keep things running smoothly, each cell has a "waste disposal system." In people with Mucolipidosis, this disposal system malfunctions.
-
Mucolipidosis is inherited, meaning it is passed down from parents to their children through genes. We all have genes that give instructions for making enzymes, which are like tiny workers inside our cells. In ML, the gene that instructs cells to make some particular enzymes is faulty, leading to a shortage or malfunction of those enzymes.
The gene responsible for Sialidosis (ML 1) is NEU1
The Gene responsible for Mucolipidosis alpha/beta is GNPTAB (Patients affected by the errors in the GNPTAB are: ML II, ML II/III Intermediate, ML III
The Gene Responsible for Mucolipidosis Gamma is GNPTG. Patients with this Gene error have ML III and is extremely rare.
-
Because of this enzyme shortage or malfunction, certain molecules called glycoproteins and glycosaminoglycans build up inside cells. Think of it like trash piling up in our city analogy. Over time, the accumulation causes problems throughout the body.
-
Mucolipidoses are rare inherited conditions where the body has trouble breaking down certain materials. There are different types of this condition, and they each have their own specific problems.
1. Mucolipidosis Type I (ML I) - Sometimes called Sialidosis:
What's going on: The body doesn't produce enough of a specific enzyme (a kind of protein that helps with chemical reactions).
What happens: Divided into Type I (mild form with late-onset) and Type II (early-onset with more severe manifestations)
Milder Form: Symptoms usually start in the teenage years. Some people may notice sudden muscle jerks, red spots in the eyes, vision problems, and balance issues.
Severe Form: Signs show up in infancy and are more intense. They might include abnormal bone shapes, facial features that look different, and intellectual challenges.
2. Mucolipidosis Type II (ML II) - Also known as I-cell disease:
What's going on: A number of enzymes are not working properly, which causes waste to build up inside cells.
What happens: Infants may show:
Facial features that look different.
Stiff joints and limited movement.
Abnormal bone shapes.
Learning and growth delays.
Problems with the heart and breathing.
Being shorter than typical.
Children with ML II pass away at a very young age.
3. Mucolipidosis III/III now known as Mucolipidosis II/III Intermediate.
What Happens - Children with this form of ML are a little bit of ML II and ML III hence them being described as Intermediate. These children have many of the symptoms of both ML II and ML III.
Some children live well into their 20’s while others will pass away at a young age.
4. Mucolipidosis Type III (ML III) - Sometimes referred to as pseudo-Hurler polydystrophy:
What's going on: It's similar to ML II, but the enzymes are only partly working, so issues are usually less severe.
What happens: Signs start to show between ages 3 and 5:
Severe bone abnormalities.
Chronic Pain
Stiff joints.
Problems with the hips and hands.
Some heart issues.
Breathing challenges.
Learning difficulties
Most people with ML III can think and learn like others their age, but they might have some physical challenges as they get older. Children with ML III live well into their 40’s but by this time are severely affected by their disease.
-
Gene therapy seeks to treat genetic disorders by introducing, altering, or replacing a DNA sequence within the cell. For Mucolipidosis, the therapeutic potential lies in targeting the faulty gene responsible for the enzymatic malfunction and replacing or repairing it.
Potential Approaches for Mucolipidosis:
Gene Replacement: This involves introducing a healthy copy of the affected gene into a patient's cells. Vectors, typically viruses that have been modified to carry human DNA, transport this healthy gene into cells. Once inside, the hope is that the cell will start to produce the functional enzyme, thereby alleviating the disease's effects.
Gene Editing: Advanced tools like CRISPR/Cas9 now offer the ability to target specific sequences of DNA within the cell and "edit" them. For Mucolipidosis, this could involve directly repairing the mutation in the individual's genome. This method holds promise for a more permanent solution, but it's also complex and comes with challenges in ensuring precision and safety.
Ex Vivo Treatment: Another strategy involves removing cells from the patient, modifying them outside the body to correct the genetic defect, and then reintroducing these modified cells back into the patient. This ensures that the genetic modifications are carried out in a controlled environment.
Challenges and Considerations
While gene therapy offers significant potential, it's crucial to note that the approach is not without challenges:
Delivery: Ensuring that the therapeutic gene reaches the required cells in sufficient quantities without affecting other cells is vital.
Immune Response: The body might recognize and attack the introduced vectors or modified cells.
Off-target Effects: Gene editing tools might inadvertently modify unintended regions of the genome, leading to unforeseen consequences.
Longevity of Treatment: It's still uncertain whether a single treatment could offer lifelong benefits or if repeated treatments would be necessary.
Adeno-associated virus 9 (AAV9): AAVs are commonly employed as vectors in gene therapy. AAVs, in general, have gained considerable attention for several reasons:
Low Immunogenicity: One of the challenges of gene therapy is the body's immune response against the viral vector. AAVs, including AAV9, tend to have a relatively low risk of triggering severe immune reactions, which makes them particularly attractive for therapeutic applications.
Broad Tissue Tropism: AAV9 has a unique ability to cross the blood-brain barrier, which means it can be used to target the central nervous system. This makes AAV9 a preferred choice for disorders that affect the brain or spinal cord.
Stable and Long-term Expression: Once the AAV delivers the therapeutic gene, it can lead to stable and long-term expression of the desired protein in targeted cells.
Non-integrating: AAVs do not generally integrate their DNA into the host genome. Instead, they mostly exist as episomes (extrachromosomal DNA circles) in the cell. This feature reduces the risk of insertional mutagenesis, where the integration of viral DNA might disrupt vital genes or activate oncogenes, potentially leading to cancer.
Safety Profile: AAVs have not been associated with any human diseases, and wild-type AAVs require co-infection with a helper virus (like adenovirus or herpes simplex virus) to replicate. This means that there's a relatively low risk of AAV causing disease on its own.
For Mucolipidosis and other lysosomal storage disorders that can affect the nervous system, the ability of AAV9 to cross the blood-brain barrier is especially significant.
-
Developing a gene therapy using AAV9 (or any other vector) is a complex and rigorous process, governed by many regulations to ensure safety and efficacy.
Here's a broad overview of the steps involved:
1. Target Identification and Validation
Disease Understanding: Identify the specific gene or genetic mutation responsible for the disease.
Therapeutic Strategy: Decide the best approach: replacing a faulty gene, silencing an overactive gene, or introducing a new gene to aid in treatment.
2. Vector Development
Choosing AAV9: AAV9 might be chosen because of its ability to target certain tissues, like the central nervous system.
Gene Packaging: The therapeutic gene is packaged into the AAV9 vector. This is often done using a process where cells in a lab are infected with a mix of modified viruses, leading them to produce the AAV9 vector containing the desired gene.
3. Preclinical Testing
In Vitro Studies: The gene therapy is tested on cells in a laboratory setting to determine its effectiveness and safety.
Animal Models: The therapy is tested on suitable animal models. This helps in understanding how the therapy behaves in a living organism, its potential benefits, and side effects.
4. Regulatory Review. Before human trials can begin, relevant regulatory bodies (e.g., the FDA in the USA) review the preclinical data. They ensure the potential benefits outweigh the risks.
5. Clinical Trials. If approved, the therapy proceeds to human trials, usually in multiple phases:
Phase 1: Assesses safety and dosage in a small group of participants.
Phase 2: Focuses on efficacy and further evaluates safety in a larger group.
Phase 3: Compares the new therapy to the current standard treatments in a much larger group of people. This phase will solidify the therapy's effectiveness, monitor side effects, and gather data for its eventual use in the broader population.
Phase 4 (Post-marketing Surveillance): Even after approval and market release, the therapy is monitored for any long-term effects or rare side effects in a diverse patient population.
6. Regulatory Approval If the clinical trials show the therapy is safe and effective, it can be submitted for final approval by regulatory bodies.
7. Accessibility and Distribution. The therapy is made accessible to patients.
-
Mucolipidoses (ML) are rare genetic disorders, and the exact prevalence varies by region and type of mucolipidosis. However, here are some general estimates:
Mucolipidosis Type I (ML I or Sialidosis): ML I is very rare, with a prevalence estimated to be less than 1 in 1,000,000 people.
Mucolipidosis Type II (ML II or I-cell disease): This is also a rare disorder. Estimates suggest that it affects approximately 1 in 100,000 to 400,000 newborns.
Mucolipidosis Type III (ML III or Pseudo-Hurler Polydystrophy): ML III is slightly more common than ML II but is still rare. The estimated prevalence is between 1 in 100,000 and 1 in 600,000 people.
It's important to note that these are rough estimates, and actual numbers might vary based on the region, population, and the methodology of data collection. Since mucolipidoses are rare disorders, they fall under the category of "orphan diseases," which can sometimes mean they are underreported or underdiagnosed. The actual global numbers might be slightly higher due to undiagnosed cases.
General FAQ (in medical terms)
-
The recent research on MLII, MLII/III and MLIII has broadened our understanding of the disease process and identified several secondary biochemical pathways altered by loss of mannose-6-phosphate biosynthesis. This information points to the potential for new modes of therapy that differ from the conventional treatments utilized in other LSDs. This is particularly important for MLII in light of the challenges associated with the standard therapeutic strategies. The failure of hematopoietic stem cell transplantation to improve MLII outcomes highlights these challenges and may indicate that enzyme replacement therapies (ERT) and gene therapies may face roadblocks in MLII.
For gene therapy, challenges may arise from the fact that the phosphotransferase is a large membrane-bound enzyme with multiple subunits and thus its expression in all affected tissues may prove difficult. Nonetheless, the availability of well characterized mouse and feline MLII models provide an encouraging platform to test the efficacy of gene therapy approaches.
Exploring the use of pharmacological agents (e.g. bisphosphonates, cathepsin inhibitors, and secondary pathway inhibitors) as potential therapies will also be a significant focus of research in the coming years. While these compounds won’t fully cure the disease or correct the underlying defect in lysosomal targeting, they can be used to slow the progression of symptoms in many tissues. Preliminary studies using pharmacological agents in the various MLII animal models including zebrafish show promise.
In conclusion, impressive progress has been made over the last decade towards the understanding of the underlying pathogenesis in this disease and many molecular aspects of the enzyme involved. New insights into the cell- and tissue-specific effects of impaired lysosomal targeting will hopefully translate into novel approaches for therapy.
-
Multiple animal models were developed and characterized for MLII in recent years including three unique mouse models and two zebrafish models. Mice that either lack GNPTAB or express a mutant form share much of the same pathology seen in patients, including neurodegeneration and abnormal bone development.
Progressive bone loss in MLII mice was shown to arise from a combination of dysfunctional bone forming cells (osteoblasts) and excessive formation of bone degrading cells (osteoclasts). This work has also demonstrated cell type-specific effects upon loss of mannose phosphorylation. For example, the finding that B cells but not T cells are affected in ML mice suggests that impaired lysosomal targeting affects cell types in different ways.
Parallel investigation of MLII zebrafish has highlighted a novel role for secreted lysosomal enzymes in cartilage and heart pathogenesis. This work demonstrates that secreted cathepsins underlie abnormal development of cartilage and cardiac tissues by altering the growth factors that regulate their development.
Suppressing this excessive cathepsin activity improves these phenotypes. Collectively, the studies in mice and zebrafish are beginning to suggest new avenues for disease treatment, such as the use of drugs that boost bone-forming cells (e.g. bisphosphonates), reduce bone-degrading cells and suppress the damaging activity of cathepsin proteases secreted outside the cell. More detailed information can be found in the following papers:
Publications:
Boonen et al. Mol Biol Cell 2011 Apr 15;22(8):1135-47. (https://www.ncbi.nlm.nih.gov/pubmed/21325625)
Vogel et al. Vet Pathol 2009 Mar;46(2):313-24. (https://www.ncbi.nlm.nih.gov/pubmed/19261645)
Kollmann et al. Brain 2012 Sep;135(Pt 9):2661-75. (https://www.ncbi.nlm.nih.gov/pubmed/22961545)
Paton et al. J Biol Chem 2014 Sep 26;289(39):26709-21. w.ncbi.nlm.nih.gov/pubmed/25107912)
Otomo et al. J Cell Biol 2015 Jan 19;208(2):171-80. (https://www.ncbi.nlm.nih.gov/pubmed/25601403)
Kollmann et al. EMBO Mol Med 2013 Dec;5(12):1871-86. (https://www.ncbi.nlm.nih.gov/pubmed/24127423)
Flanagan-Steet et al. J Bone Miner Res 2016 31(3):535. (https://www.ncbi.nlm.nih.gov/pubmed/26404503)
Petrey et al. Dis Model Mech 2012 Mar;5(2):177-90. (https://www.ncbi.nlm.nih.gov/pubmed/22046029)
Flanagan-Steet et al. Am J Pathol 2009 175(5):2063-75. (https://www.ncbi.nlm.nih.gov/pubmed/19834066)
-
Our understanding of how GlcNAc-1-phosphotransferase is made in the cell and the significance of conserved domains with this enzyme has greatly expanded over the last ten years. Cell-based studies have identified the key protease (site 1 protease) responsible for cleaving the GNPTAB gene product into active alpha and beta subunits and revealed how different domains and subunits of the phosphotransferase enzyme function to add mannose 6-phosphate residues to lysosomal enzymes. From this work we now understand which domains of the GNPTAB gene product are important for catalysis and which are needed to specifically recognize lysosomal hydrolases.
Defining the function of these domains and how the subunits of the phosphotransferase enzyme interact with each other provides the opportunity to study how missense or point mutations within the alpha/beta (encoded by GNPTAB) and gamma (encoded by GNPTG) subunits affect the biosynthesis, transport and activity of the enzyme.
Collectively, this information has helped explain how different patient mutations (i.e. genotype) correlate with unique disease symptoms and severities. In some cases, specific mutations (e.g. K4Q) have been linked to clinically distinct forms of the disease. Significant new insight into the function of the gamma subunit has also been gained over the last five years. Studies have demonstrated that the trafficking and activity of this subunit can be impacted by its proteolytic processing in certain cell types as well as its post-translational modification. Still others studies have demonstrated that some missense mutations in GNPTG can cause misfolding of this subunit. Lastly, attempts to engineer the phosphotransferase enzyme have successfully demonstrated that certain variants can actually increase the mannose phosphorylation of both lysosomal and non-lysosomal substrates. More detailed information can be found in the following papers:
Publications:
Liu L et al. Mol Ther Methods Clin Dev. 201729;5:59-65 (https://www.ncbi.nlm.nih.gov/pubmed/28480305)
van Meel et al. J Biol Chem 2016 Apr 8;291(15):8295-307 (https://www.ncbi.nlm.nih.gov/pubmed/26833567)
Qian et al. J Biol Chem 2015 Jan 30;290(5):3045-56 (https://www.ncbi.nlm.nih.gov/pubmed/25505245)
De Pace et al. Hum Mutat 2014 Mar;35(3):368-76 (https://www.ncbi.nlm.nih.gov/pubmed/24375680)
Qian et al. Proc Natl Acad Sci 2013 Jun 18;110:10246-51 (https://www.ncbi.nlm.nih.gov/pubmed/23733939)
Velho et al. Hum Mol Genet 2015 Jun 15;24(12):3497-505 (https://www.ncbi.nlm.nih.gov/pubmed/25788519)
Marschner et al. Science 2011 Jul 1;333(6038):87-90 (https://www.ncbi.nlm.nih.gov/pubmed/21719679)
van Meel et al. Proc Natl Acad Sci 2014 Mar 111:3532-7 (https://www.ncbi.nlm.nih.gov/pubmed/24550498)
Leroy et al. Eur J Hum Genet 2014 May;22(5):594-601. (https://www.ncbi.nlm.nih.gov/pubmed/24045841)
Pohl et al. J Biol Chem 2010 Jul 30;285(31):23936-44 (https://www.ncbi.nlm.nih.gov/pubmed/20489197)
Encarnacao et al. J Biol Chem 2011 Feb;286(7):5311-8 (https://www.ncbi.nlm.nih.gov/pubmed/21173149)
Van Meel et al. Hum Mutat Jul;37(7):623-6 (https://www.ncbi.nlm.nih.gov/pubmed/27038293
FAQ about Sialidosis (ML I)
-
Sialidosis, also known as mucolipidosis I, is a neurosomatic lysosomal storage disease belonging to the group of the glycoproteinoses. The disease is inherited as an autosomal recessive trait and is caused by genetic lesions at the NEU1 gene encoding the lysosomal sialidase, neuraminidase 1 or NEU1. This enzyme is essential for initiating the degradation of glycoproteins containing the sugar sialic acid (SIA) at the end of their glycan chains. Thus, defective or deficient activity of NEU1 leads to the buildup of sialylated glycoproteins in lysosomes and other subcellular sides.
The excessive and progressive accumulation of non-digested or only partially digested SIA-containing metabolites in many cells of the body is pathognomonic of the disease, and at the basis of the disease pathogenesis. Sialidosis affects primarily cells of the reticuloendothelial system, so tissues and organs of epithelial origin show the first and most acute signs of the disease.
The patients present with two distinct clinical conditions:
Sialidosis type I (normomorphic) is also referred to as “cherry red spot - myoclonus syndrome” because gradually reduced visual acuity and twitching of the muscles are often the only discernible symptoms at least initially. Normal intellectual capacity, late presentation and long survival are characteristics of these forms and explain why patients with type I sialidosis go undiagnosed for many years or are misdiagnosed. However, the occurrence of the aforementioned features combined with abnormal secretion of high molecular weight sialyloligosaccharides in urine and low NEU1 activity in white blood cells or fibroblast could help in making the diagnosis of most of these cases. Thus, this type of sialidosis patients might be more numerous than previously thought.
Sialidosis type II (dysmorphic) is the severe, neuropathic form of the disease. Three subtypes are recognized within this group, depending on the time of onset and severity of the symptoms: congenital or hydropic with onset in utero, infantile with onset between birth and 12 months of age, juvenile with onset past 2 years of age. The clinical presentation includes coarse face, enlargement of spleen and liver, vertebral deformity, dysostosis multiplex, neurological involvement.
In general, the severity and penetrance of specific phenotypic abnormalities correlate with the type and combination of NEU1 mutations and the levels of residual enzyme activity. More than 40 NEU1 disease-causing mutations have been identified in type I and type II sialidosis patients. They are mostly missense mutations leading to single amino acid substitutions.
Publications:
Seyrantepe et al 2003 https://pubmed.ncbi.nlm.nih.gov/14517945/;
Caciotti et al 2009 https://www.ncbi.nlm.nih.gov/pubmed/19568825;
d’Azzo et al 2015 http://www.ncbi.nlm.nih.gov/pubmed/26949572;
Franceschetti et al 2016 https://www.ncbi.nlm.nih.gov/pubmed/27621198)
Neeraja K et al 2020 https://pubmed.ncbi.nlm.nih.gov/33121223)
-
The nature and biochemical characteristics of NEU1 have hindered the development of suitable therapies for sialidosis. The enzyme by itself tends to aggregate, it is highly immunogenic, and it is catalytically inactive unless bound to its auxiliary chaperone PPCA. Nonetheless, the strict dependence of NEU1 on PPCA for catalytic activation and stability may turn advantageous for therapeutic purposes. This is because NEU1 residual activity in fibroblasts from several type I patients could be increased by increasing the levels of available PPCA. This indirect therapeutic approach may be conceivable for patients carrying NEU1 missense mutations that permit its interaction with PPCA. In this regard, both AAV-PPCA–mediated gene therapy and PPCA-ERT that hopefully will become available for the treatment of patients with galactosialidosis in the near future, could, in principle, be extended to the treatment of at least some patients with type I sialidosis. If this will become an optional therapy for these patients, its successful implementation and the identification of eligible patients will require the coordinated effort of expert clinicians, basic scientists, pharmaceutical companies, and most importantly family organizations and patient advocacy groups.
Publications:
Hu et al 2012 http://www.ncbi.nlm.nih.gov/pubmed/22008912
Bonten et al 2013 http://www.ncbi.nlm.nih.gov/pubmed/23770387
In absence of other therapeutic approaches readily available for patients, the repurposing of FDA approved compounds, over the counter medicines as well as dietary supplements may ameliorate the quality of life of patients with sialidosis. This approach has been recently used in sialidosis patients’ cells where it was shown that NEU1 activity increased, following administration of several therapeutics. Interestingly, dietary supplementation of betaine, a natural amino acid derivative, in mouse models with residual NEU1 activity, mimicking type I sialidosis, increased the levels of mutant NEU1 and resolved the oligosacchariduria.
Mosca et al 2020 https://pubmed.ncbi.nlm.nih.gov/32143456)
In conclusion, despite the rarity and complexity of silaidosis, remarkable progress has been made over the last decade towards the understanding of the underlying pathogenesis in this disease. If the bench work will continue at this pace, I am inclined to predict that additional new functions for NEU1 will be uncovered that might open new possibilities for alternative therapies. In this respect I believe that the most helpful hints on the roads to such discoveries often come from the sialidosis patients themselves and their families. Their insightful and courageous drive is a daily encouragement for us scientists to move ahead and keep looking forward.
-
NEU1 is ubiquitously but differentially expressed in different tissues and cell types. The enzyme is catalytically active and stable in lysosomes when in complex with two other enzymes: the protective protein/cathepsin A (PPCA) and β-galactosidase. NEU1 interaction with PPCA is essential for maintaining its activity; hence, PPCA functions as a canonical chaperone/auxiliary protein for NEU1. Extensive biochemical studies have been done on complex formation and mode of assembly of NEU1 and PPCA; although a full understanding of the way these proteins interact awaits the resolution of the 3 dimensional structure of the complex. Detailed information about these studies can be found in the following recent papers:
Bonten et al 2009 http://www.ncbi.nlm.nih.gov/pubmed/1966647;
Bonten et al 2014 http://www.ncbi.nlm.nih.gov/pubmed/24337808)
-
Neu1 KO mouse was generated and characterized in early 2000. Total loss of Neu1 activity in these mice is compatible with life, but they develop a severe and progressive condition closely similar to type II sialidosis, and have a short lifespan (5-7 months) compared to wild type mice. KO mice present with hallmarks of the disease already at birth, including oligosacchariduria and extensive lysosomal vacuolation of cells in virtually all systemic organs and the nervous system. Epithelia, reticulo-endothelia and histiocytes/macrophages are the first cell types to get affected.
The main pathologic features that recapitulate those seen in patients are growth retardation, time-dependent enlargement of the spleen, diffuse edema (buildup of fluid), spine abnormalities and neurodegeneration. The sialidosis mouse model has proven valuable to study the basis of disease pathogenesis downstream of Neu1 deficiency and consequent impaired processing or degradation of substrates that are target of the enzyme in vivo.
de Geest et al 2002 http://www.ncbi.nlm.nih.gov/pubmed/12023988
These studies have uncovered new functions of lysosomal NEU1 in basic physiological processes that go beyond its degradative capacity, and, when deregulated, contribute to the expression and severity of specific phenotypic alterations known to occur in sialidosis. For example, we now can explain, at least in part, the mechanism, controlled by Neu1, that underlie progressive spleen enlargement, hearing loss, muscle atrophy and neurodegeneration in the Neu1 KO mouse and likely in patients.
Some of the cellular and extracellular changes have been attributed to the capacity, and loss thereof, of NEU1 to keep in check the process called lysosomal exocytosis. The way NEU1 does it is through the processing of the SIAs on one of the enzyme’ substrates, the lysosomal membrane protein LAMP1. When NEU1 is deficient, LAMP1 remains unprocessed and this causes an abnormal number of lysosomes to move to and dock at the membrane surrounding the cells and eventually to fuse with such membrane and discharge their contents extracellularly. This continuous, excessive discharge of potentially harmful products from the lysosomes eventually affects the balance between the intracellular and extracellular composite of molecules that maintain tissue and organ physiology. Detailed information about this research and its implication for sialidosis can be found in:
Yogalingam et al 2008 http://www.ncbi.nlm.nih.gov/pubmed/18606142;
Zanoteli et al 2010 http://www.ncbi.nlm.nih.gov/pubmed/20388541;
Wu et al 2010 http://www.ncbi.nlm.nih.gov/pubmed/19857571;
Annunziata et al 2013 http://www.ncbi.nlm.nih.gov/pubmed/24225533
Contact us
If you have any questions or comments, please don’t hesitate to reach out using the contact form. You can also reach us by email.
Email
info@curemucolipidosis.org